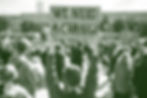
Only two decades ago, some scientists were skeptical that renewables could occupy more than 20% of our electricity grid.
In the U.S., that milestone was hit in 2020, and over the next 30 years, plans outlined by the International Energy Agency place the world at net-zero emissions by 2050 in order to combat global warming.
A huge part of reaching net zero is getting to 100% clean electricity and doing it cost-effectively.
Over the course of our renewable energy quest, we’ve hit targets across green electricity. In 2020, over 80% of all new electricity capacity was renewable.
Clean energy businesses now employ more people worldwide than oil and gas. And in the first half of 2022, renewables generated over one-quarter of all power used in the US. In the early months of the year, solar generation alone grew by 36% compared to last year.
Solar and wind have doubled production between 2015 and 2021. As we hit all of the right targets, scientists know clearly how we will get to a 90% renewable energy grid. But the last 10% – the bullseye on the dartboard – is a little hazy.
Researchers refer to “the last 10%” as a nonlinear spike in costs to build and operate the power system seen in the last generation of renewables.
The “last 10% challenge” is nothing new. When meeting the last increment of demand, there’s always a hitch.
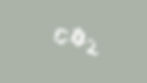
When it comes to our global quest to reach net-zero and 100% clean electricity by 2050, researchers have a pretty good understanding of how to cost-effectively get to the 90th percentile. However, not only is there little agreement about the best pathway to the last 10% but achieving the milestone gets quite expensive.
A recent study published in the journal Joule gives insight into achieving the last lap, identifying six possible approaches.
These approaches range from increasing our reliance on wind and solar to scaling up carbon capture and removal, to building out energy storage using hydrogen to going nuclear. In the paper, the researchers – based at the National Renewable Energy Laboratory (NREL) – compare economic factors and unique challenges associated with each strategy.
The researchers emphasize that the last 10% plan will be a combination of multiple approaches rather than just one. While our current situation, and many energy systems still a long way from requiring the last 10%, preliminary research is necessary so that when we get there, options are available.
“A 100 percent carbon-free power system will require a portfolio of resources,” the lead researcher Trieu Mai told Inside Climate. “But humility is needed to accept that we don’t know what the optimal mix to solving the last 10 percent” is going to be.
The rise in costs associated with the last 10% is largely driven by a seasonal mismatch between the timing of variable renewable energy generation and demand. Meeting peak demand is challenging and expensive for any technology but in the case of renewable energy, addressing seasonal mismatch may require newer technologies that have yet to be deployed on a large scale.
"None of the strategies are perfect, and a lot of uncertainty remains, but the study highlights key challenges with the last 10% and examines all the major technology options," Mai said in a statement, "More research and development will be important to move closer to a clear solution for the last 10% and progress the United States toward a decarbonized power sector."
The ideal solution is going to have 3 characteristics.
It must have resource adequacy, or the ability to supply enough electricity in the right locations. When a power system has resource adequacy, it can keep the lights on even in events of extreme weather like those seen across the Caribbean and North Atlantic over the last week. The solution also must have low capital costs, since it won't be used as often as other renewables. And the solution will need to rely on broadly, currently available resources so it can be deployed at scale.

Each of the six strategies NREL outlines has the potential to hit all three targets.
1. Existing renewables like solar and wind with short-term energy storage
The first strategy relies on existing tech. In this option, variable renewable energy like wind and solar are expanded to meet demand during daily stressful periods on the grid. Battery storage would be used to fill hourly supply gaps and curtail excess energy.
A benefit of this option is that low costs are already available, but the researchers note a downside could be the site constraints for installing more wind and solar farms over time, as land use for solar and wind has led to conflicts in local communities in the past.
However, wind and solar are no longer limited to land. New research and funding into alternatives such as floating wind farms and solar farms could prove useful. Just last week Biden’s executive actions launched funding into offshore floating wind farms on U.S. coasts.
Still, there’s the storage problem. Combining battery storage with offshore wind has been explored by startups like Dutch Ocean Graze which developed a battery that stores the turbines’ energy underwater, Scotland’s Equinor which developed the first offshore storage solution in 2018, and both the Danish group Orsted and America’s Bay State Wind who have been exploring battery integration to make wind power more reliable across seasons.
2. Geothermal, hydrogen, and biomass, oh my!
Another possible approach to the last 10% would be using less ubiquitous renewables like geothermal, hydropower, and biomass.
Already likely to play all-important roles in the zero-emission power sector, these technologies do not rely on the variability of solar and wind. Able to operate around the clock, the researchers suggest they can overcome seasonal mismatch. However, the con of this option is low resource availability, especially at locations with high electricity demand. For example, geothermal has limits on where it can be built, hydropower is vulnerable to falling water levels. Therefore these may only prove useful in select regions.
Additionally, compared to solar and wind, these renewables have a relatively high capital cost. Compared to the other two, biomass-based generation is lower. Still, there are uncertainties and constraints on a steady and sustainable feedstock supply and the cost of biomass conversion.
3. Nuclear and fossil fuels with carbon capture
Nuclear is already used in modern-day carbon-free electricity. Carbon capture and storage (CSS), the process of capturing carbon emitted from fossil fuel plants and storing it underground, has been widely cited, along with nuclear, as an important resource in a decarbonized electricity grid. (NREL reported nuclear and carbon capture together because of common traits like their 24/7 capacity and high initial costs.)
While the study notes that CCS has yet to be deployed at scale and is only operating in small numbers if fossil fuel plants can be retrofitted with the technology, it has the potential to power the last 10%.
Still, this strategy comes with a slew of challenges from the limited recent deployment of CCS to its cost uncertainties. There are environmental and security considerations around including fossil fuel plants in our last 10% plan and employing nuclear which could have high capital costs along with existing safety concerns.
Startups like FootPrint Coalition portfolio company, Commonwealth Fusion Systems, are working to solve the problems associated with nuclear fusion. Commonwealth, specifically, uses high-temperature superconducting magnets to generate the force to contain the plasma created in the company’s power plant. According to the International Energy Agency, nuclear power could be 10% of the world's energy supply by 2050, potentially solving the “last 10%” challenge.
4. Seasonal storage using hydrogen
As NREL reports, hydrogen or hydrogen-derived fuels are currently the most promising option for seasonal storage. Seasonal storage refers to using electricity to produce a storable fuel that can be used for power generation for up to, entire seasons of the year.
According to NREL’s precious research, hydrogen has the “greatest potential” for storage due to its low costs, expected cost-competitiveness, and most importantly, the power efficiency it’s able to produce.
Converting hydrogen to electricity can be done using fuel cells or combustion technologies, which are already being done by startups globally. With this option, energy companies would use renewable energy to produce hydrogen from water, and then the hydrogen could be stored in vast tanks. When energy demand meets its peak, hydrogen would be released as a secret weapon to power a gas turbine or fuel cell.
Despite its inexpensiveness, ability to be stored in large quantities, and the number of companies already working on the technology, hydrogen storage is far away from being ready for market leading to speculation around its supply and delivery infrastructure. Still, if it can be deployed at scale, it could play the vast role that natural gas plants play in our energy grid today.
5. Carbon dioxide removal
At present, carbon dioxide removal, or CDR, offsets emissions from carbon-emitting power generation technologies by drawing down atmospheric carbon. The difference between this and carbon capture and storage is that CSS captures carbon from smokestacks like a coal-fired power plant or a cement factory and sequesters it underground, while CDR takes it directly from the air. This term covers a variety of strategies such as direct air capture or planting trees, which naturally remove carbon dioxide.
This specific option is unique because it leverages other strategies to support resource adequacy on the grid. Plus, in the event a major part of reducing emissions in the electricity sector, it will be essential in other hard-to-decarbonize industries like steel, cement, and chemical.
Compared to the other options, however, CDR is extremely expensive and because it has yet to be deployed on a global scale, future costs remain uncertain.
6. Reducing electricity demand
The last option feels quite obvious, but is easier said than done. Compared to the other strategies, this advocates for energy conservation, reducing both the demand for electricity and naturally, the costs.
As the study reports, reducing electricity during peak times of demand reduces stress on the grid and avoids investments into new peaking capacity. After all, it's cheaper to conserve a kilo-watt hour than generate one, no matter which of the above 5 methods is used. Other pros include making the grid more reliable and less susceptible to interruption.
However, it’d be an understatement to say energy conservation is complicated on both the policymaker and consumer levels. Moreover, employing this type of strategy for the last 10% challenge requires resources readily available over multi-day periods in case of extreme events or natural disasters.
The last 10% challenge is nothing new, and it will likely take a combination of these strategies in order to get to a 100% carbon-free grid. But the question remains: which strategies are in the magic equation, and to what extent?
"Given current technology costs and readiness, significant emissions reductions can occur through accelerated deployment of wind, solar, diurnal storage, transmission, and other renewable energy technologies," Paul Denholm, NREL analyst and co-author of the study said in a statement.
"Other technologies could also play a big role if they become cost competitive and widely available. We will continue to study these possible solutions, but for now, the pathway to about 90% carbon-free electricity is increasingly clear. After all, getting to 100% requires first achieving 90%."